The faculty members with primary research interests in Cosmology are Charles Bennett, Marc Kamionkowski, Tobias Marriage, Brice Ménard, and Adam Riess. Below we describe a small subsample of ongoing projects and exciting recent results.
Research in cosmology takes place along a variety of theoretical and observational/experimental directions, led by members of Johns Hopkins’ Cosmology Discovery Group. Prof. Charles Bennett is Principal Investigator (PI) of the Wilkinson Microwave Anisotropy Probe (WMAP), a NASA satellite which operated from 2001 to 2010 and returned the most detailed maps ever made of the tiny fluctuations in the cosmological microwave background. From a careful study of their character, his team has been able — for the first time ever — to measure accurately the age of the Universe (13.7 Gyr), as well as provide much tighter constraints on many other important cosmological parameters, such as the curvature of the Universe, its mean density, the Hubble constant, etc., opening up the era of precision cosmology. Our effort in this area was augmented with arrival of Prof. Tobias Marriage, who played a major part in building the Atacama Cosmology Telescope, a mm-wave telescope 5000m above sea-level in Chile, studying fine angular-scale structure in the microwave background. The group is engaged in constructing Cosmology Large Angular Scale Surveyor (CLASS), a cosmic microwave background polarization experiment funded by the National Science Foundation. The goal is to detect for the first time the expected gravitational waves from inflation via the induced B-mode polarization pattern in the cosmic microwave background. Once built, the instrument will be observing in the Atacama desert in Northern Chile, one of the driest places on the planet.
A false color image of the intensity of the CMB; typical fluctuation scales Delta T/T are ~10^{-5}
Graduate students J.Eimer and L.Zeng and Prof. Bennett (in the middle) photographed through the largest wire polarizer ever built — Cosmology Large Angular Scale Surveyor.
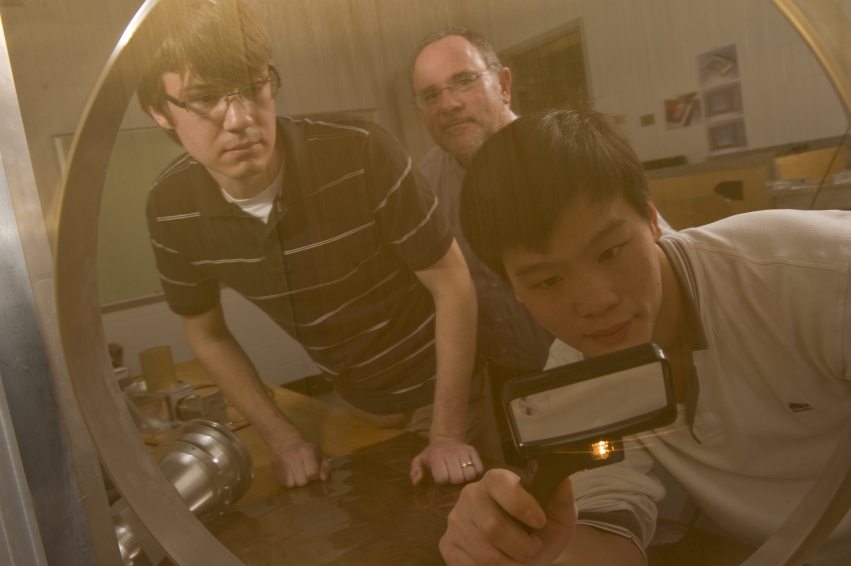
Arguably the biggest cosmological surprise encountered is the very large contribution to the expansion of the Universe due to the mysterious “Dark Energy”. Through his use of high-redshift supernovae to measure the cosmological distance scale, Prof. Adam Riess has contributed more to this discovery than perhaps any other single person. Prof. Riess received the 2011 Nobel Prize in physics for the discovery of the accelerated expansion of the Universe.
Ongoing research in Riess’s group focuses on precise measurements of the expansion of the cosmos starting with the local universe and continuing out beyond 10 billion light years. By measuring the speed at which the universe expands at different epochs across cosmic time, they hope to deduce the reason behind the universe’s recent acceleration – the nature of dark energy. Measuring cosmic expansion requires the distance to galaxies and the speed at which they move away from us. The most direct method is to measure distance using a “standard candle”, an astrophysical object or event of known luminosity, for example Type Ia supernovae which occur when a white dwarf that was once about the size of our sun gains matter from a second, nearby star until it reaches a point at which it begins to collapse under its own gravity and ignites a thermonuclear detonation. The Pan-STARRS project of which JHU is a full member discovered over 5,000 supernovae of all types by imaging ~70 square degrees of sky regularly over four years. Out of these 5,000, Prof. Riess’ group found that ~1,200 are Type Ia supernovae that can measure accurate cosmological distances. This is the largest current sample of Type Ia supernovae and can be used to measure dark energy better than ever before.
Distance modulus as a function of redshift (left) and constraints on the dark energy equation of state (w) and matter density of the universe (ΩM) (right) from two Pan-STARRS supernova samples. The smaller sample (Rest et al. 2014) uses Type Ia supernovae that had their type confirmed with spectroscopy. The larger sample (blue) has much more statistical leverage on dark energy, but requires a newly developed technique developed by graduate student David Jones and Prof. Riess to separate the Type Ia supernovae from non-Ia supernovae.
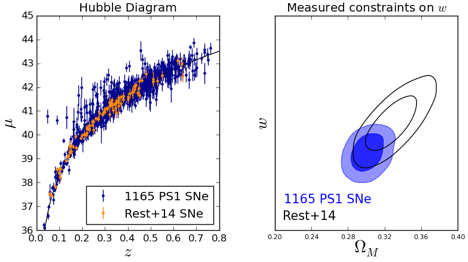
Prof. Marc Kamionkowski works on a variety of subjects in cosmology and particle astrophysics. Much of his recent research has aimed to develop new ways to use cosmic microwave background data to constrain fundamental physics and to probe the early Universe. He has also been pursuing the development of analogous tests that can be carried out with large-scale-structure data and, more recently, what ultimately may be learned from 21-centimeter probes of atomic hydrogen. Prof. Kamionkowski has a longstanding interest in exploring ideas for particle dark matter and methods for its detection. He is also interested in exploring questions involving dark energy, galaxy formation and the physics of galaxy clusters, neutrino physics and astrophysics, and stellar astrophysics.
Six different types of distortions to an otherwise isotropic two-point correlation function. Such distortions may be imprinted on the cosmological mass distribution if the inflaton responsible for primordial perturbations couples to some new tensor (left two columns), scalar (middle two columns), or vector field (right two columns).
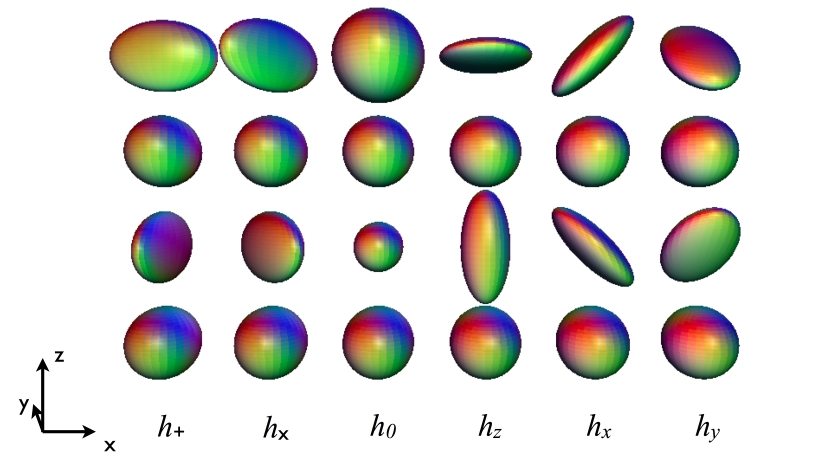
Prof. Brice Ménard is analyzing massive datasets from the SDSS-I, II and III surveys to learn about formation and evolution of galaxies, to map out the dark matter distribution with gravitational lensing and to study the distribution of tenuous matter filling the space between cosmic structures.
Left: Visualization of about 200,000 absorption line systems detected in the SDSS quasar spectra cataloged by JHU postdoc Dr. Guangtun Zhu and Prof. Brice Ménard. Right: Spatial distribution of cool gas around star-forming and passive galaxies obtained by graduate student Ting-Wen Lan, Prof. Menard and Dr. Zhu using the SDSS dataset. They found more gas around star forming galaxies than around passive ones. This difference is likely due to gas outflows driven by galactic winds.
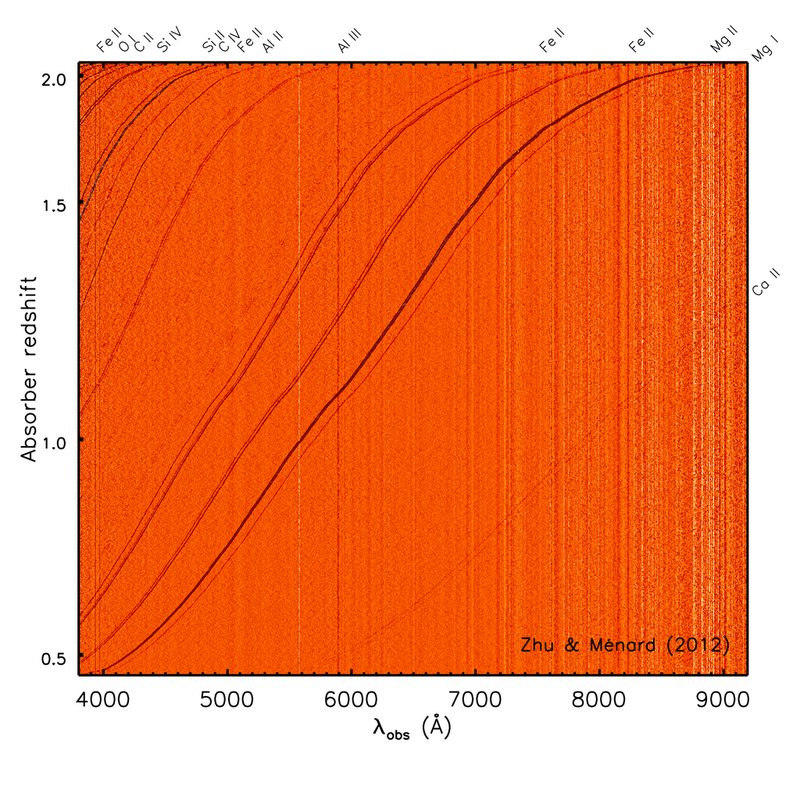
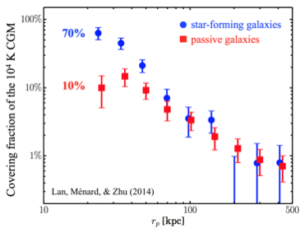