Self-assembly and self-organization in cell biology
In our research group we study dynamical systems in biology, both in and out of equilibrium. We are interested in addressing questions related to control of self-assembly in the cell, which has to occur at the right time and place for proper function. We are especially fascinated by clathrin-mediated endocytosis and viral exit from cells. Our multidisciplinary group tackles questions both on principles and design of self-assembling systems, as well as biological function in cells. To study these complex systems, we simulate networks of many interacting components, and construct simplified models that are amenable to analytical theory, with foundations in statistical mechanics. We use and develop modeling techniques from physics, chemistry, and engineering, including molecular models for thermodynamics, reaction-diffusion models for stochastic dynamics in cells, and continuum models for coupling to mechanics. We collaborate frequently with experimental groups.
Spatial and temporal dynamics of vesicle formation in clathrin-mediated endocytosis
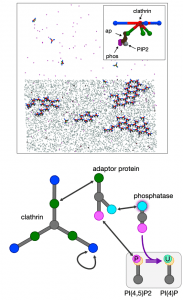
Clathrin-mediated endocytosis is occurring persistently within our cells, performing transport of essential nutrients such as iron into our cells, and clearing receptors at our neural synapse. It involves coordinated assembly of dozens of different protein components, and viral infection or neurological disorders can result from its dysfunction. Our research has shown how localization to the membrane can dramatically enhance assembly of even very weakly interacting partners via dimensionality reduction from 3D to 2D, which effectively concentrates components and promotes binding. At the systems level, we have quantified how the stoichiometry of the network of protein and lipid components involved in CME can sensitively tune the speed or success of vesicle formation, highlighting again the central role of membrane localization in triggering protein assembly. With reaction-diffusion simulations, we have quantified how essential lipid phosphatases are capable of driving disassembly of the membrane-bound assemblies. Ongoing research in our lab is focused on predicting the timescales of binding in and on the 2D membrane, mechanical feedback between membrane binding and bending, and timescales of receptor and cargo uptake. We are broadly interested in how key features of dimensionality and component stoichiometry control assembly in CME, virion formation, and other cellular pathways.
Control of assembly on membranes
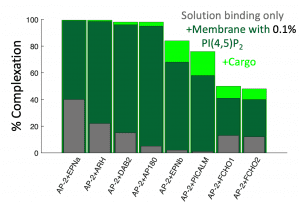
In many cellular pathways, including endocytosis, viral exit, receptor-mediated signaling, and cell adhesion, proteins must localize to membranes as well as interact with other proteins. For proteins that can bind to one another both in solution and on the membrane, the membrane offers a reduced search space (in nearly all cases) that will thus concentrate proteins and promote more binding. We have developed theory to quantify how significant a role this dimensionality reduction plays in controlling protein interactions. Quantifying this behavior is critical to understanding assembly on surfaces because 2D localization will strengthen binding reactions regardless of whether additional factors, such as curvature generation or membrane microdomains, also influence binding. Because the localization of specific proteins to membranes also can induce membrane curvature and bending, we are quantifying how perturbations to membrane mechanics can feedback into the stability of subsequent protein-membrane interactions.
Network biology: mechanisms to models
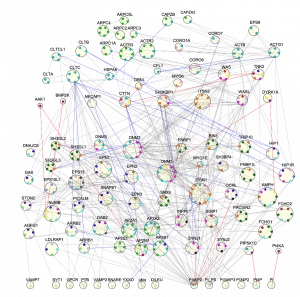
A biological process like clathrin-mediated endocytosis involves 50-80 different types of cytoplasmic proteins, lipids, and cargo receptors, with copy numbers of each varying from millions (clathrin) to hundreds (auxilin). Because constructing accurate dynamical models of such large-scale networks is both expensive and dependent on hundreds of parameters, we develop models to infer function from integrating network structure and copy numbers. To infer function, protein networks must include binding interfaces, capturing both shared and specialized binding interactions. We have shown how these interface-resolved network have specific network motifs that support high binding specificity, with sub-optimal motifs largely indicating regulatory control of interactions. To integrate copy-numbers, we have developed a simple model of binding stoichiometry that can be optimized across a large network. With this model, we can rapidly evaluate which proteins are in significant excess/shortage of copies relative to the full global network of partners. We find robust trends in which proteins are sub- or super-stoichiometry both in terms of network structure (i.e. hubs) and protein function (i.e. enzymes), across three mammalian cell type. Combined with removal of nodes or ‘knock-downs’, we can use this approach to construct predictive hypotheses about the role of key cargo and adaptor protein knockdowns on endocytic function.
Methods development in computer simulations of cell biochemistry and biology
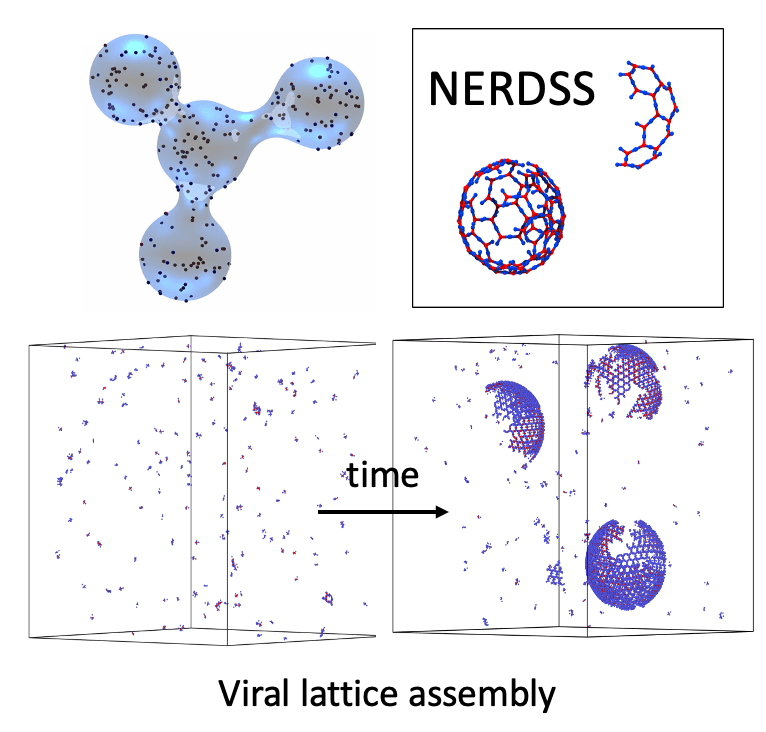
The dynamics of self-assembly in the cell are challenging to simulate due to their dependence on fast molecular motion and slow collective dynamics, along with coupling to energy-consuming reactions. The reaction-diffusion methods developed in our lab enable simulations inaccessible to existing software tools, with broad applications in cell biology and engineering design. Our NERDSS software uses our Free-propagator Reweighting (FPR) algorithms to correctly capture reaction dynamics in solution, on surfaces, and as recruited to surfaces, with the ability to build in coarse molecular structure and orientation into binding reactions. We are also developing methods for coupling the biochemistry of these processes with the mechanics of membrane deformation and budding. We compare these new methods with existing stochastic and deterministic spatial and non-spatial techniques, along with theory, for careful validation and to establish the regimes where spatial effects or diffusion are important sources for controlling reaction dynamics.